Pharmaceuticals
CPhI Worldwide 2021 Annual Report: Innovation in manufacturing will fuel the next decade of industry growth 3rd November 2021
By Editorial Team for CPhI Worldwide Special - Bikash Chatterjee, Chief Executive Officer,Pharmatech Associates—a USP Company
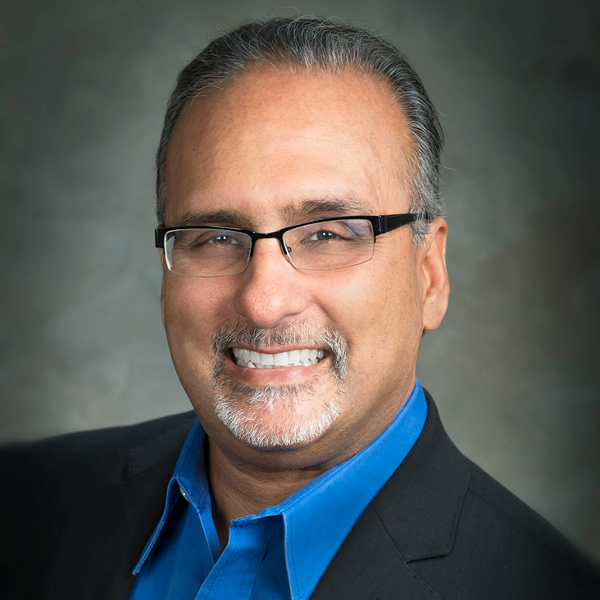
This is the third of our exclusive articles adapted from the 2021 CPhI Worldwide Annual Report, which is released at the event (November 9-11, 2021). The full report will be available for download at cphi–online.com
As the world struggles to emerge from the COVID-19 pandemic we have caught a glimpse of what our industry can accomplish when properly motivated. For the past decade we have seen both the pharmaceutical industry and the Food and Drug Administration embrace innovation in nearly every facet of the drug and device development process. We have also seen that theengine driving these innovations is no longer the exclusive domain of large pharma and biotech.
Looking at the more than 3,0001 drug programmes in Phase 3 clinical programmes cited in the congressional budget office report on pharmaceutical research and development, more than 70 per cent come from companies with revenues of $500 million or less. Since 2009, more than one third of new drug approvals by the FDA have gone to drug sponsors with revenues less than $100 million1. Correspondingly, for the first time in decades, speciality drugs top the ranking based on retail spending, whereas classes in which the best-selling drugs are now available in generic form rank lower than they did just ten years ago1.
Even if one were to ignore these trends, our industry’s ability to innovate, test, scale up, and mass produce highly effective vaccines against the COVID-19 virus illustrates our aptitude to harness latent innovation and execute action. Close collaboration and communication between academia, industry, regulators and government policymakers has never been stronger, and will become a foundation for future innovations in our industry for years to come.
Innovation in manufacturing: the ripple effect
The innovations we have witnessed in the past decades—particularly for rare diseases—provide hope to millions of people while creating new challenges in the manufacturing and scale-up of cost-effective drug therapies. The FDA expects to approve between 10-20 new Biologics License Applications (BLAs) a year for the next four years in the areas of cell and gene therapy alone.1 But these therapies are costly for manufacturers and patients alike. To realize the long-term sustainability of many new drug modalities and therapies, industry must rethink its approach to manufacturing, quality control, and the corresponding path to overall drug quality.
The impact of advanced manufacturing technologies goes beyond the simple implementation of new technology. Broad acceleration in adoption will be achieved, in part, through focused intensive development between academia and industry. We have been slow to adopt paradigm-shifting approaches and technologies because of the industry’s aversion to perceived risk, but the pandemic thrusted telemedicine and decentralized clinical trial design to the forefront after they had languished for years as an interesting theoretical discussion. Our renewed collective focus on supply chain resiliency, on national preparedness, and the need to drive business performance in a post-pandemic market place are catalysts for modernization that will extend throughout manufacturing and the entire value chain, thereby reducing industry risk aversion with a ripple effect upon traditional manufacturing practices. Advanced technologies will affect many future manufacturing operations; some of these key areas are shown in Figure 1.
Figure 1. Advanced manufacturing innovation areas.
Process Analytical Technology (PAT)
Whether a drug sponsor is content to stick with the current hybrid state of batch manufacturing for its drug substance and/or drug product, or is seeking to migrate to a continuous process, the ability to gain rapid insight into the state of the material within each unit operation is central to having confidence the material manufactured possesses the correct critical quality attributes (CQA) for it to be safe and efficacious. With the FDA’s paradigm-shifting guidance in 20042encouraging scientific understanding and intelligent application of risk management as the basis for defining drug quality, the ability for drug manufacturers to implement solutions that allow them to see and react to process information in real time has been the goal of the FDA and other regulatory bodies. Process analytical technology (PAT)strives to understand processes by monitoring the critical process parameters (CPP) that affect a product’s CQA. The goal is to monitor and potentially control them—in as close to real time as possible—through either in-line or on-line measurement sensors, thus ensuring the process remains within its process design space.
Process Analytical Chemistry (PAC) Technologies
To fully realize the benefits of a PAT implementation it needs to be combined with process analytical chemistry (PAC) tools to measure the characteristics of the process that have been defined as critical material attributes (CMA, or critical process parameters, CPP). Several PAC technologies have emerged as the most promising and practical for PAT implementation. For active pharmaceutical ingredient (API) manufacturing, Raman spectroscopy has proven to be a very effective optical method to determine molecular structure and chemical composition. Raman spectroscopy as a tool for API analysis has been described for many applications, including polymorph identification, quantitative analysis, in-situ crystallization monitoring, real-time release testing, pharmaceutical unit operations, and process-induced transformations. In addition to identifying isolated polymorphic forms, mixtures of forms can be analyzed and quantified. In-line Raman spectroscopy can control critical process parameters, as it enables real-time process corrections, and ensures consistent production of the correct API form.3 Near-infrared spectroscopy (NIR) is an efficient surrogate to high-pressure liquid chromatography (HPLC). Another effective measurement technology is energy dispersive X-ray diffraction (EDXRD)—particularly to measure the characteristics of powders— and has shown to have adequate sensitivity and resolution to detect critical material property changes during processing.
Standards for PAT and PAC methods
As PAT adoption grows, it is likely that the International Council for Harmonisation (ICH) or regulatory compendia will institute practice standards to guide organizations in the qualification of PAT and PAC methods. The application of PAT is completely unique to the material characteristics and specific equipment and sensor technology being applied, and the framework and criteria for qualification should be universal. I would look to compendia organizations such as the United States Pharmacopeia (USP) and the British Pharmacopeia (BP) to lead the way in establishing practice standards that greatly demystify the qualification component of PAT implementations.
The ultimate goal of PAT is to minimize or eliminate the time lost with release testing of intermediate unit operations and the final product. The ability to shrink this time within the overall value stream of the process affects the overall cost of goods, trickling down to inventory carrying costs, plant capacity, and market response capability. One manufacturing site that implemented a combination of in-line and at-line release was able to reduce its product release cycle time by greater than 80 per cent, lessening the normal in-process and release testing cycle time from 30 days to five days4.
One of the greatest impediments to PAT adoption has been the ability to gather, curate and analyze large amounts of data. Today the solutions for handling and analyzing big data are mature. With the right financial motivation, coming from downward pricing pressure, addressing drug shortages, or government-sponsored reshoring initiatives, the advantages of targeted PAT implementation should drive much broader implementation in the near future.
Real time release (RTR)
The requirements for real time release (RTR) have been defined for most major markets. The European Commission issued Annex 17 Guidance addressing real time release and parametric release and the FDA has addressed this issue in a number of guidances that convey which process controls together with in-line monitoring of the manufacturing process can provide greater assurance of product quality compared to just a traditional testing of the final materials. As the industry gains comfort and familiarity with PAT, the adoption of an RTR strategy will become more commonplace.
Advanced manufacturing –Continuous manufacturing
The FDA defines5 advanced manufacturing as a collective term for new manufacturing approaches and technologies that can improve drug quality, address shortages of medicines, and reduce time-to-market. By this rationale, advanced manufacturing is all about innovation and includes integrating novel technological approaches, or using established techniques in a new or innovative way, or applying production methods in a new domain where there are no defined best practices or experience.
At the centre of the advanced manufacturing narrative is the potential for the industry to move from batch to continuous manufacturing. One might see continuous manufacturing as a way to provide a more consistent and cost-effective process framework for manufacturing small-molecule drugs, including both the active pharmaceutical ingredient (API) and the final drug product (DP). Enthusiasm for continuous manufacturing has been rekindled by the US Government’s interest in supply chain resiliency and in reshoring to address the nation’s unbalanced dependency on China and India for API and finished DPs for essential medicines.
Batch vs. continuous
It is important to state that step-by-step batch processing to describe traditional small-molecule drug product manufacturing is an inaccurate description. Most oral solid dose manufacturing processes today utilize a mix of batch, semi-batch, semi-continuous, and continuous unit operations. For example, if one were to look closely at a traditional oral solid dose process, only the drying and blending steps are truly batch processes. Regardless of whether doing high-shear or fluid bed granulation the process always involves adding granulation fluid to the powder, so at best these are semi-batch processes. The same goes for coating tablets. In milling and tableting the operation continuously processes material as a batch, so these can be considered semi-continuous processes. Therefore, a more accurate description of the principles underlying the current state is a hybrid approach.
The International Committee on Harmonization (ICH) accounted for this in the recently published ICH Q13 draft guideline3pertaining to pharmaceutical continuous manufacturing (PCM) recognizing that PCM can be applied to some—or all—unit operations in a manufacturing process. PCM modes include both hybrid batch continuous applications of continuous manufacturing to continuous manufacturing across the entire API and drug product manufacturing process.
Advocates for pharmaceutical continuous manufacturing cite the many potential benefits a continuous manufacturing process brings. These benefits include lower facility capital costs, smaller process footprint requirements, the ability to more accurately identify and segregate potentially discrepant material, and the ultimate potential benefit of real time release (RTR) that removes or greatly reduces the time required for in-process and release testing of the final API or DP. Critics argue that the return on investment to change over to PCM is poor, given the added complexity of process development, testing capability, regulatory compliance and the recall risk from larger batch sizes.
Material selection and characterization considerations
To grasp the complexity of designing and setting up a continuous process one need only look at the primary regulatory characteristic that distinguishes a continuous process from a batch process—the residence time distribution (RTD). TheRTD characterizes the material as it moves through the process and is a probability distribution that describes the amount of time a mass or fluid component remains in a process step.
The shape of the RTD reflects the degree of axial dispersion or backmixing within that system and the RTD is dependent upon several factors such as input material attributes including mass flow rates, process parameters, equipment design and operation.
It is important to understand how the RTD varies over both the control and design space for each unit operation. This information serves as a basis for material traceability and for defining the RTD. While both batch and continuous processes are dependent upon the characteristics of the material, continuous manufacturing characterization is significantly more complex, since the output of one unit operation has the potential to be the input material for the next process without the benefit of sampling and testing. Characterization data can be used to develop in-silico models that describe the behavior of materials within the unit operation. Often this is the first glimpse into how the material characteristics and process parameters interact.
We have stated that adoption of continuous manufacturing will be bolstered by strong government support as part of the reshoring effort to secure supply chain resiliency for medicines that are essential to US national security and the wellbeing of the nation. Yet, the characterization and analysis techniques we describe have value for today’s hybrid batch processes.The enhanced process efficiency and commensurately lower cost of goods will be a boon to drug sponsors and the national agenda as we set about establishing lower cost health care in the US.
Automation strategies
The capacity to realize reductions in cost of goods, increased product quality and flexible manufacturing response capability is a direct by-product of a firm’s automation strategy. With downward pricing pressures an omnipresent reality, automation is likely to be at the heart of identifying cost centres that negatively impact the cost of goods or a firm’s ability to meet market demand. Looking at the industry today, we note several trends that are likely to grow in importance in terms of automating manufacturing operations:
Robots and cobots
Industrial robots have been deployed as islands of automation for decades. Operations that require manual human intervention, such as material loading and unloading, palletizing post-packaging, or other repetitive tasks, have long utilized robotic solutions. Robots are deployed successfully in the laboratory to provide 24-hour processing capability for highly repetitive and time-consuming testing, such as chromatographic and dissolution testing.Collaborative robots, or ‘cobots’, are improving efficiency in pharmaceutical research, drug production, and quality control. They can be programmed for a variety of tasks, relocated easily, and can start working quickly and they can be mounted above workspaces to save valuable floor space for humans and equipment in small labs. Cobots free human operators and analysts from fatiguing repetitive tasks and allow them to focus on higher-complexity work.
Cloud computing
Adopting cloud-hosted over server-based solutions has had a large impact on manufacturing operations. Because it is encrypted, cloud-hosted data is extremely secure and enables pharma companies to meet relevant compliance and regulatory requirements by providing enhanced visibility and control over their business processes. The cloud computing or software as a service (SaaS) model has many other advantages: lower capital investment, significantly smaller GxP IT infrastructure support requirements, and greater flexibility in addressing data management requirements. Moving to a cloud platform allows manufacturers to use new types of production systems, from 3D printing and high-performance computing (HPC) to the Internet of Things (IoT) and industrial robots. With well defined qualification and architecture frameworks to address data integrity and security considerations, cloud computing will continue to be a backbone of the manufacturing framework for many years to come.
21st Century supply chain management
Adding automation to supply chain applications affects efficiency, capacity, and overall cost of goods for a drug sponsor. Typically, optimized supply chains are characterized as having less than 50 per cent inventory holdings, and three times faster cash-to-cash cycle compared to under-optimized supply chains.6
Automated storage andretrieval systems (ASRS) can increase the accuracy of picking and staging material for processing to 99 percent. Studieshave shown that having a human pick an order is more than 50 per cent of the total picking time.
Blockchain technologies have the potential for faster delivery of safe medications while meeting government reporting requirements at the same time and are key to critical, effective traceability through better data sharing. Optimizing the total value chain will become a core component of any business strategy by leveraging a combination of automation and cloud computing solutions.
Digital integration
The factory of the future will control a number of digital solutions to provide information quickly and accurately wherever it is needed throughout the operation. The use of extended reality (XR) is already demonstrating its value in modern manufacturing operations. Extended reality subsumes virtual reality, augmented reality and mixed reality. Put simply, the focus is on merging the physical with the virtual world. Virtual reality (VR) is a fully immersive simulated experience that replaces the real world with a virtual environment at a 1:1 scale. Augmented reality (AR) adds a digital layer of information onto real surroundings, providing new ways of interacting with reality. And finally, mixed reality (MR) is just as it sounds – a combination of these, where real-world and digital objects interact.
Pharma is using VR to make full-scale digital facility environments that allow people to walk through a facility before it exists in reality, to spot any flow errors, to test equipment, or examine critical and difficult design tasks.
Training and Operational Support
Our industry has long grappled with achieving more effective training of personnel. Virtual reality allows people to review procedures and execute them through simulation in a realistic virtual environment, without the need for physical equipment. VR training can also allow personnel to understand the consequences of incorrect behaviour by simulating changes in airflow and contamination in a safe, virtual environment.
This is advantageous because many pharma companies have global operations. Duplicating staff in multiple sites can be costly when the quality and experience of staff varies based upon the facility location. Augmented reality allows remote staff to direct and participate in critical support activities by live streaming with subject matter experts (SMEs) in multiple locations— without having to travel—saving time and money while assuring valuable training to all site personnel.
Artificial Intelligence (AI)
Slowly but surely, AI is making its way into the pharmaceutical sector, opening new possibilities and breaking new competitive ground for ingenious pharmaceutical companies ready to leverage leading-edge technologies.
AI is a broad term that covers areas that include replicating human cognitive processes (i.e., symbolic logic) and machine learning (ML). Using machine learning allows for accurate predictions, classifications, and identification of patterns – in the same way as the human neural network, but much more efficiently and on a much bigger scale.
AI model training
One area that is frequently cited is that the amount of data required to train a machine-learning or deep-learning model is extremely high, and pharma doesn’t typically generate the same levels of data as the telecom or semiconductor industries do. In reality the amount of data required depends both on the complexity of the problem to solve and on the complexity of the chosen algorithm. For solutions that are data-constrained, there are data augmentation techniques that can be used to refine and optimize a model.
Manufacturing applications of AI
Equipment maintenance is one area in pharmaceutical manufacturing that is not data-constrained. AI has been very successfully applied to equipment monitoring to build models that predict equipment failure, in part because it is very easy to gather millions of data points when continuously measuring equipment vibrations. AI is used to develop equipment duty cycles and proactive preventative maintenance procedures that increase the overall equipment effectiveness (OEE) of manufacturing operations, avoid catastrophic equipment failures, and reduce waste and discrepant material.
An area where machine learning has been very effective is in supply chain management and inventory management to improve forecast accuracy, demand management, inventory management and manufacturing scheduling. Look for ML applications to be more broadly deployed across the supply chain as organizations look to drive business performance amid continued pricing pressure.
Cost of Poor Quality (COPQ)
The cost of poor quality, or COPQ, is an important theme that will likely play a much bigger role in pharma’s evaluation of the effectiveness of operational improvements. COPQ covers both the direct and indirect costs associated with defects generated by a process. Such costs vary depending on how far the product or service goes along the process before being detected. If one can address a defect or process excursion early in a process, the cost is diminished. If one has to remediate a quality event at the customer level, it is much more expensive and, in our industry, the regulatory governance can dramatically raise the financial exposure if it results in a recall or enforcement action. Every time there is an excursion to the normal process that results in discrepant material, or in additional sampling, testing and evaluation, an organization’s quality management system is engaged and that has a cascade effect upon the bottom line due to the associated additional inventory, investigation, and lost revenue costs.
COPQ consists of three basic cost categories: appraisal costs, internal failure costs, and external failure costs. Appraisal costs are the costs associated with sampling and testing material for conformance to a predefined specification. Internal failure costs pertain to defects identified within an operation before shipping to the customer. External costs are associated with defects found by the customer. PAT represents a forward-thinking solution to minimize appraisal costs by measuring product in-line or at-line within the process and not incurring addition labour and risk by sampling and testing after processing. Layered on top of these three is cost of prevention. These are the costs incurred in the management and execution of your Quality Management System (QMS). Organizations that track and manage to COPQ will realize significant acceleration in both cost reduction and overall business performance.
Conclusion
Whether continuous manufacturing is to become a major development within the overall pharmaceutical manufacturing market in the future is difficult to predict. It is more certain that initiatives such as reshoring, and a sharpened focus on ensuring the integrity of the strategic national stockpile (SNS), will be a springboard for the development and refinement of advanced manufacturing technologies.
For industry, the chief benefit from broader adoption of such approaches is to take advantage of lower cost of goods by reducing waste and the cost of poor quality (COPQ), and that in itself may increase the industry’s comfort with principles such as realtime release (RTR). Integrating machine learning into advanced manufacturing programmes has the potential to drive the efficiency ofindividual unit operations and boost overall business operations. This will result in more stable supply chains and potentially lower costs all around.
In a market likely to continue to encounter consistent downward pricing pressures, the adoption of these elements will allow manufacturers to lower their costs and still manage a profit as they provide improved, higher -quality drug therapies to patients— this is a win-win in anyone’s book.
REFERENCES
1. Research and Development in the Pharmaceutical Industry, Congressional Budget Office, April 2021, https://www.cbo.gov/publication/57126
2. Health and Human Services, US Food and Drug Administration, Pharmaceutical CGMPs for 21st Century- A Risk Based Approach, Final Report, September 2004, https://www.fda.gov/about-fda/center-drug-evaluation-and-research-cder/pharmaceutical-quality-21st-century-risk-based-approach-progress-report
3. K.A, Esmonde-White et al., Raman Spectroscopy as a process analytical technology for pharmaceutical manufacturing and bioprocessing, Analytical and Bioanalytical Chemistry, 409, 637-649 (2017), https://link.springer.com/article/10.1007/s00216-016-9824-1
4. Maximilien, J., 2019, ControlStrategy Approaches inContinuous Manufacturing of Oral Solid Dosage Forms, Drug Delivery Formulation Summit, March 13, 2019, Berlin Germany
5. Advanced Manufacturing, Food and Drug Administration website, https://www.fda.gov/emergency-preparedness-and-response/mcm-issues/advanced-manufacturing
6. Lowe H., How Supply Chain Management is Changing the Landscape, Select Hub, 2021,https://www.selecthub.com/supply-chain-management/supply-chain-automation/
The ninth edition of the CPhI Annual Report – a comprehensive report analyzing key trends and innovations in pharma – was commissioned by Informa Markets and will be available for download from www.cphi-online.com during CPhI Worldwide, which is being held at Fiera Milano, Milan, Italy, November 9-11, 2021.