Pharmaceuticals
Have you got the physical form of your API under control? 14th October 2021
By Dr Jonathan Loughrey & Professor Tom Moody
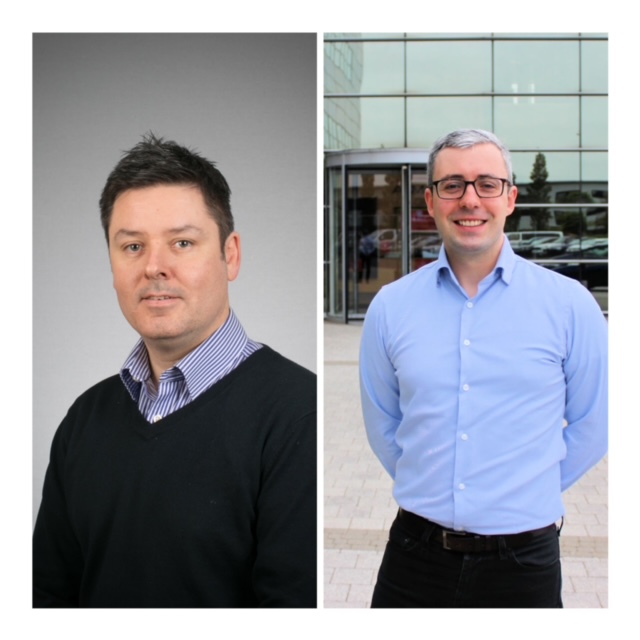
Selection and isolation of the correct stable polymorph is a key driver for confidence in your API product, with crystallization as the key to success. Starting from historical considerations and moving through to modern techniques for creating the required final dosage form, Dr Jonathan Loughrey and Professor Tom Moody of Almac Group describe strategies to ensure that a robust process for a crystalline product is achieved.
Identifying, selecting and protecting the correct polymorphic form of an API (free forms, salts, and co-crystals), is a critical decision that has a significant effect not only on commencement of any scale-up and product manufacture but also on the commercial deliverables and protection of product IP. Moreover, it creates confidence that the product API will remain consistent throughout formulation development and clinical trials, an essential trait for any existing or new drug product.
Fine-tuning API properties
Once the polymorphic form of the API (both small molecule and peptide) has been identified and selected, preformulation and drug product development teams are on hand to fine-tune the physiochemical properties of the drug substance. This is critical to maximize drug bioavailability and stability and this information should be fed seamlessly back to crystallization chemists and engineers who are able to develop robust and scaleable crystallization processes. Reliable preparation of the targeted form with specific solid form properties (e.g. flowability, particle size, and morphology) is essential. Feedback loops between teams is a critical feature of modern solid form development and as the industry generally turns to APIs with increased complexity, selecting and isolating the correct polymorphic form of an API is becoming more challenging with modern strategies and techniques being developed. This narrative explores such key considerations for API crystallization, polymorph selection, and how modern advances in equipment and techniques ensure isolation of the desired solid form, which in turn can be manufactured with confidence.
Robust and reliable procedure
Designing and engineering a robust and reliable crystallization procedure is a critical step in the selective isolation and purification of an active pharmaceutical ingredient (API) or high-value chemical target, most commonly with a molecular weight between 100 and 3000 Daltons. Without a robust isolation procedure, minor changes to the processing conditions may have a disastrous impact on product quality and subsequent downstream activities. As a result, the isolation of crystalline API is commonplace within the pharmaceutical industry and it is estimated that more than 90% of APIs are isolated as a crystalline product.[i],[ii] Pharmaceutical crystallization process development has been accelerated as a topic of discussion due to numerous high-profile cases related to the solid form of an API; the isolation of (S)-(-)-thalidomide instead of (R)-(+)-thalidomide,[iii],[iv] the discovery of ritonavir form II 18 months after commercial launch,[v],[vi] and the recall of ritigotine (Neupro) in 2008 upon identification of a new polymorph during stability storage.[vii] These examples highlight the importance of understanding and controlling the solid form of an API to ensure safety to the end user.
Most commonly isolated in batch processes from stirred tank reactors,1 the controlled crystallization of an API offers numerous advantages. The most obvious is that the purity of the API is enhanced by holding any process impurities in solution and selectively precipitating the API. A recall of Ranitidine by the FDA in 2019 due to unacceptable levels of the known carcinogen N-nitrosodimethylamine illustrates this importance of selectively isolating the API to control unwanted impurities that may have severe detrimental health effects on the patient.[i] Further advantages of API crystallization include fine-tuning of the particle shape, size, and crystal morphology and, crucially, isolation of the desired polymorphic form.
Polymorph selection
Selecting the most suitable polymorphic form of an API for further development is the first step towards achieving a robust and scaleable crystallization procedure. Typically, studies to probe and understand the polymorphic landscape are completed relatively early in the development cycle (i.e. at a preclinical stage) because changes to the isolated polymorphic form are well documented to give rise to different physiochemical properties and aqueous solubilities.[ii]
To avoid such an issue, the most stable (thermodynamic) form of the API is commonly sought via polymorph screening experiments which evaluate the isolation of different solid forms from wide ranging solvated and solvent-free conditions. Examples of such conditions include common temperature cycling / ageing within different solvent systems, sub-ambient cooling experiments, anti-solvent addition experiments or evaporation, to techniques that favour metastable forms including polymer templating, sonication, sublimation and milling. Achieving an understanding of the polymorphic landscape is critical to guarantee that the stability of the final product is understood and that it behaves predictably upon isolation and storage in a warehouse for an extended period of time. It is also important to understand stability, as it is known that polymorphs can change when formulated with particular excipients, or even in the presence of common plastics or polymers in medical devices.[iii]
Once the solid-form properties of the API are understood and the polymorphic form selected for further development, teams of specialist crystallization chemists and chemical engineers continue the development towards reproducible preparation on a larger scale. To provide a crystallization process that provides material exhibiting desirable quality attributes (i.e. high purity, high recovery, desirable morphology, good bulk density, and excellent flowability), a deep understanding of the solubility of the API (both representative crude material and the desired polymorphic form) within suitable solvent systems is required.
Solvents that present low toxic potential as identified by the ICH are generally preferred to avoid complicated washing and drying studies upon isolation.[iv]The width of the metastable zone with respect to temperature or solvent / anti-solvent composition may then be determined to provide critical information on the degree of supersaturation available within the solvent system that may be tuned to control crystal growth. This is then translated into a choice of solvent or solvent / anti-solvent mixture for the final isolation steps, which are normally designed in temperature-controlled glass lined reactors with overhead agitation, and experimentally guided using predictive computational fluid dynamics software (e.g. DynoChem®) and optimized using experimental design software packages (e.g. JMP) (see Figure 1). However, where the crystals are fragile, Oslo-type crystallizers, continuous forced circulation and draft tube baffle crystallizers may be used to avoid significant mechanical attrition.[v],[vi] Post-isolation modification technologies, e.g. wet or jet milling, may also be used to further fine-tune particle properties.[vii]
Development challenges
Despite being the preferred method of crystallization and isolation,1 individual unit operations do add significant time to API development. Common challenges faced using batch crystallizers include the control of supersaturation within a given solvent system, appropriate use of seed crystals,[viii] determining the concentration of the API within mixed solvent systems, retention of the desired polymorphic form, and the formation of crystalline fines and their impact on crystal size distribution (CSD) via secondary nucleation. A drive towards increasing efficiency within pharmaceutical manufacture has emerged in recent years by introducing continuous production and automated processes to achieve consistent product quality and a higher production rate.[ix],[x],[xi]Of these continuous crystallization technologies, the most commonly used continuous systems are mixed-suspension mixed-product removal (MSMPR) crystallizers and tubular / plug flow crystallizers (PFC), laminar-flow tubular crystallizers (LFTC), coiled flow inverter (CFI) crystallizers, segmented/slug flow crystallizers, and oscillatory baffled crystallizers (COBC).[xii] These continuous crystallizers may be coupled with automated feedback loops to maintain supersaturation and provide a consistent quality product. Such feedback loops are provided by process analytical technologies (PAT), most commonly ATR-FTIR or ATR-UV/vis probes to monitor solute concentration, focused beam reflectance modules (FBRM) and on-line microscopy to monitor precipitation rate and particle size or morphology, and Raman probes, which provide polymorphic identification. When coupled with model-based predictive controls using statistical and mathematical modelling to plan, optimize and monitor the crystallization process, product with the desired critical quality attributes (CQAs) may be achieved incorporating both quality by design (QbD) and quality by control (QbC) approaches.[xiii],[xiv],[xv]
Control and efficiency
Despite being the oldest and most commonly used method to isolate an API, the crystallization unit operation has matured significantly since the 1990s. A general drive towards control in recent years has also been coupled with efficiency, exemplified by the approval of continuous manufacturing processes for the APIs Orkambi and Darunavir in 2015 and 2016, respectively. Based on the advantages of continuous crystallization, it is entirely reasonable to query why batch crystallization dominates the industry, therefore, the next decade will undoubtedly see a shift towards continuous processing as companies and manufacturing plants update their capabilities and follow the strong support FDA has shown for continuous processing technologies.[xvi],[xvii]
In conclusion
There is no doubt that control of impurities and physical forms of new APIs are at the forefront of innovators’ minds. However, it does require time and investment to get this right for a given API and, in some cases, can be put to the side to ensure timelines are met. This is a risky strategy. As projects are accelerated to move through trials at lightning speeds, it is essential not to forget about physical form control for both the drug product and drug substance stages, as control is essential to ensure success. Innovators do not want to have any surprises late into development causing clinical delays. Have you got your solid form under control? It is time to get your API under control!
Meet the authors
Dr Jonathan Loughrey graduated from the University of York with a MChem Degree in July 2010 and obtained a PhD in organic chemistry from the University of Leeds in December 2013. After postdoctoral appointments in the UK and US, he returned to the UK to join a small pharmaceutical contract research laboratory in 2014, ultimately moving up the ladder to lead the company’s global solid form screening business function. Dr Loughrey joined Almac Sciences in June 2021 and is currently Physical Sciences Manager, specializing in solid form screening, process crystallization development, and preformulation development of small molecules and peptides, and has been involved with the solid form development of more than 200 pharmaceutical targets over the past five years.
Professor Tom Moody graduated from Queen’s University Belfast with a 1st Class BSc (Hons) in chemistry in June 1998 before returning to gain a PhD in Physical Organic Chemistry in December 2001. He has also completed a Masters in Business Degree, graduating with distinction in July 2007, specializing in business strategy. His work has earned him numerous accolades and he is co-author and author of more than 90 publications and patents. Professor Moody is currently VP Technology Development and Commercialisation for Arran Chemical Company and Almac and works in the area of Chemistry & Biocatalysis and its application towards the synthesis of chiral molecules, metabolites and labelled compounds. He is responsible for managing a multi-disciplinary team of both chemists and biologists to obtain commercially useful biocatalysts and develop their intended applications, biocatalytic processes having been developed from mg to tonne scale and including development of fermentation processes to yield the desired biocatalyst. Professor Moody has been a scientific leader and problem solver in more than 50 commercial projects in the past three years and acts as a consultant in the area of biocatalyst development for pharmaceutical and biotech companies. He is also an Honorary Professor at Queen’s University Belfast in the area of biocatalysis.
[i] Chen, J.; Sarma, B.; Evans, J. M. B.; Myerson, A.S. Pharmaceutical Crystallization.Cryst. Growth Des. 2011, 11(4), 887-895.
[ii] Yu, Z. Q.; Chew, J. W.; Chow, P. S.; Tan, R. B. H. Recent Advances in Crystallization Control: An industrial Perspective.Chem. Eng. Res. Des. 2007, 85 (7), 893-905.
[iii]D’Amato, R.J.; Loughnan, M.S.; Flynn, E.; Folkman, J. Thalidomide is an Inhibitor of Angiogenesis.
Proc Natl Acad Sci USA,1994, 91 (9), 4082-4085.
[iv]Wnendt S.; Finkam M.; Winter W.; Ossig, J.; Raabe, G.; Zwingenberger, K. Enantioselective Inhibition of TNF-α Release by Thalidomide and Thalidomide-Analogues Chirality, 1996, 8 (5), 390-396.
[v] Bauer, J. F.; Saleki-Gerhardt, A.; Narayanan B. A.; Chemburkar S. R.; Patel K. M.; Spiwek H. O.; Bauer, P. E.; Allen, K. A., inventors; Abbott Laboratories, assignee. Polymorph of a Pharmaceutical. United States patent US 8193367 B2. 2012 Jun 5.
[vi]Bauer, J.; Spanton, S.; Henry, R.; Quick, J.; Dziki, W.; Porter, W.; Morris, J.Ritonavir: An Extraordinary Example of Conformational Polymorphism Pharm. Res., 2001, 18, 859-866.
[vii] Rietveld, I. B.; Céolin, R. Rotigotine: Unexpected Polymorphism with Predictable Overall Monotropic Behaviour. J. Pharm. Sci.,2015, 104 (12), 4117-4122.
[i] https://www.fda.gov/news-events/press-announcements/statement-alerting-patients-and-health-care-professionals-ndma-found-samples-ranitidine. Accessed 24 September 2021.
[ii] Newman, A. Specialized Solid Form Screening Techniques. Org. Process Res. Dev. 2013, 17 (3), 457-471.
[iii]Saunier, J.; Mazel, V.; Paris C.; Yagoubi, N. Plolymorphism of Irganox: Discovery of New Forms and Direct Characterization of the Polymorphs on a Medical Device by Raman Microspectroscopy. Eur. J. Pharm. Biopharm. 2010, 75(3), 443–450.
[iv]ICH Guideline Q3C (R8) on Impurities: Guideline for Residual Solvents. Published 20 May 2021.
[v]Lu, J.; Li, Y.P.; Wang, J.; Ren, G. B.; Rohani, S.; Ching, C.B. Crystallization of an Active Pharmaceutical Ingredient That Oils Out.Separ. Purif. Tech, 2012, 96, 1-6.
[vi]Paroli, F. Industrial crystallizers design and control. Chianese, A.; Kramer, H.J.(Eds.),Industrial Crystallization Process Monitoring and Control,Wiley-VCH, Weinheim (2012), 203-224.
[vii] A. Eren, A.; Szilagyi, B.; Quon, J. L.; Papageorgiou, C.D.; Nagy, Z. K. Experimental Investigation of an Integrated Crystallization and Wet-Milling System with Temperature Cycling to Control the Size and Aspect Ratio of Needle-Shaped Pharmaceutical Crystals. Cryst. Growth Des. 2021, 21(7), 3981-3993.
[viii]Zhang, F.; Shan, B.; Wang, Y.; Zhu, Z.; Yu, Z.-Q.; Ma, C. Y. Progress and Opportunities for Utilizing Seeding Techniques in Crystallization Processes. Org. Process Res. Dev. 2021, 25, 1496-1511.
[ix]Mascia, S.; Heider, P.L.; Zhang, H.; Lakerveld, R.; Benyahia, B., Barton, P.I., Braatz, R. D.; Cooney, C. L.; Evans, H. M. B.; Jamison, T. F.; Jensen, K. F.; Myerson, A. S.; Trout, B. L.End-to-End Continuous Manufacturing of Pharmaceuticals: Integrated Synthesis, Purification, and Final Dosage Formation.Angew Chem Int Ed, 2013, 52 (47), 12359-12363.
[x]Myerson A. S.; Krumme M.; Nasr M.; Thomas H.; Braatz R. D. Control Systems Engineering in Continuous Pharmaceutical Manufacturing. May 20–21, 2014 Continuous Manufacturing Symposium.J Pharm Sci, 2015, 104 (3), 832-839.
[xi]Adamo, A.; Beingessner R. L.; Behnam, M.; Chen J.; Jamison, T. F.; Jensen, K. F; Monbaliu, J.-C. M.; Myerson, A. S.; Revalor, E. M.; Snead, D. R.; Stelzer, T.; Weeranoppanant, N.; Wong, S. Y.; Zhang, P. On-Demand Continuous-Flow Production of Pharmaceuticals in a Compact, Reconfigurable System.Science, 2016, 352 (6281), 61-67.
[xii]Orehek, J.; Teslić, D.; Likozar, B. Continuous Crystallization Processes in Pharmaceutical Manufacturing: A Review. Org. Process Res. Dev., 2021,25 (1), 16-42.
[xiii] Abbas, A.; Romagnoli, J. A. Multiscale Modeling, Simulation and Validation of Batch Cooling Crystallization. Sep. Purif. Technol. 2007, 53, 153−163.
[xiv] Nagy, Z. K.; Fevotte, G.; Kramer, H.; Simon, L. L. Recent Advances in the Monitoring, Modelling and Control of Crystallization Systems. Chem. Eng. Res. Des. 2013, 91 (10), 1903−1922.
[xv] Schmidt, B.; Patel, J.; Ricard, F. X.; Brechtelsbauer, C. M.; Lewis, N. Application of Process Modelling Tools in the Scale-Up of Pharmaceutical Crystallisation Processes. Org. Process Res. Dev. 2004, 8 (6), 998−1008.
[xvi] Yang, X.; Acevedo, D.; Mohammad, A.; Pavurala, N.; Wu, H.; Brayton, A. L.; Shaw, R. A.; Goldman, M. J.; He, F.; Li, S.; Fisher, R. J.; O’Connor, T. F.; Cruz, C. N. Risk Considerations on Developing a Continuous Crystallization System for Carbamazepine. Org. Process Res. Dev. 2017, 21 (7), 1021−1033.
[xvii] Lee, S. L.; O’Connor, T. F.; Yang, X.; Cruz, C. N.; Chatterjee, S.; Madurawe, R. D.; Moore, C. M. V.; Yu, L. X.; Woodcock, J. Modernizing Pharmaceutical Manufacturing: From Batch to Continuous Production. J. Pharm. Innov. 2015, 10 (3), 191−199.