Agrochemicals
Multi-component crystallization of pyrimethanil: a case-study 29th August 2018
By Suzanne Buttar, Thierry Bonnaud, Osama Suleiman, Kate Wittering, Joe Harris and Chloe Bartlet
Johnson Matthey’s scientists explain how multi-component crystallization can improve dissolution rate and crystal morphology by
Johnson Matthey’s scientists explain how multi-component crystallization can improve dissolution rate and crystal morphology by considering pyrimethanil as a case study.
One of the most important aspects of any organic molecule is its solid state crystalline form. This is because it governs fundamental physiochemical properties that impact the effectiveness of compounds across a range of industries. However, it is possible through solid state manipulation, to engineer a crystalline form to deliver a desired property for a specific application.
Multi-component crystallization, which includes salt formation and co-crystallization, is often explored in the agrochemical and pharmaceutical industries. It involves taking a given small molecule, the active ingredient (AI), and engineering a multi-component crystal to optimize properties such as bioavailability, stability and melting point. For instance, altering the form via multi-component crystallization could increase the rate of dissolution, an important property for pharmaceuticals as it controls how fast a molecule can be dissolved in the body prior to bloodstream absorption.
Extensive research has been performed at Johnson Matthey to showcase the benefits of multi-component optimization and the improvements made to multiple pharmaceuticals and agrochemicals. Here, we showcase how multi-component crystallization can improve dissolution rate and crystal morphology by considering the agrochemical pyrimethanil.
Pyrimethanil, a functional example
Pyrimethanil is a small organic molecule used as a broad-spectrum fungicide. It has also been the focal point of multiple literature studies investigating salt formation and co-crystallization owing to its available functional groups.
The first step in multi-component crystallization is to assess the parent compound, measuring the pKa and identifying the structural motifs and potential bonding interactions present. A range of appropriate coformers and/or counterions can then be selected. Optimization can be achieved using prior knowledge of successful multi-component crystal formation from databases of known salts and cocrystals. This process can also highlight coformers that may impact the target property – in this case dissolution rate.
For pyrimethanil, examination identified dimer interactions, similar to those observed in carboxylic acid molecules. Therefore, one of the early investigations was to crystallize pyrimethanil with a series of carboxylic acids and other coformers with motifs known to form dimers. Seventeen different cocrystal systems were investigated, and it was found that the in-vitro dissolution profile of pyrimethanil could be preferentially increased or decreased depending on the chosen counterion/coformer molecule (Figure 1).
What makes this process challenging is its unpredictability. Both salt and cocrystal formation will have a significant impact on the crystal packing, which results in a new crystalline form, changing the physiochemical properties of the solid. However, even with prior knowledge of other multi-component crystals, it is currently not possible to reliably predict the crystal structure and thus the physicochemical properties. This means all combinations must be individually synthesized, characterized and tested to assess the impact on the desired property.
Furthermore, salts and cocrystals, themselves can exhibit different crystal forms and therefore different properties. One example investigated further was the multi-component crystallization of pyrimethanil with L-tartaric acid, which crystallizes in two stoichiometries: mono and hemi. Owing to the importance of crystal structure in both pharmaceuticals and agrochemicals, optimized crystallization processes must be designed that preferentially crystallize the most developable form.
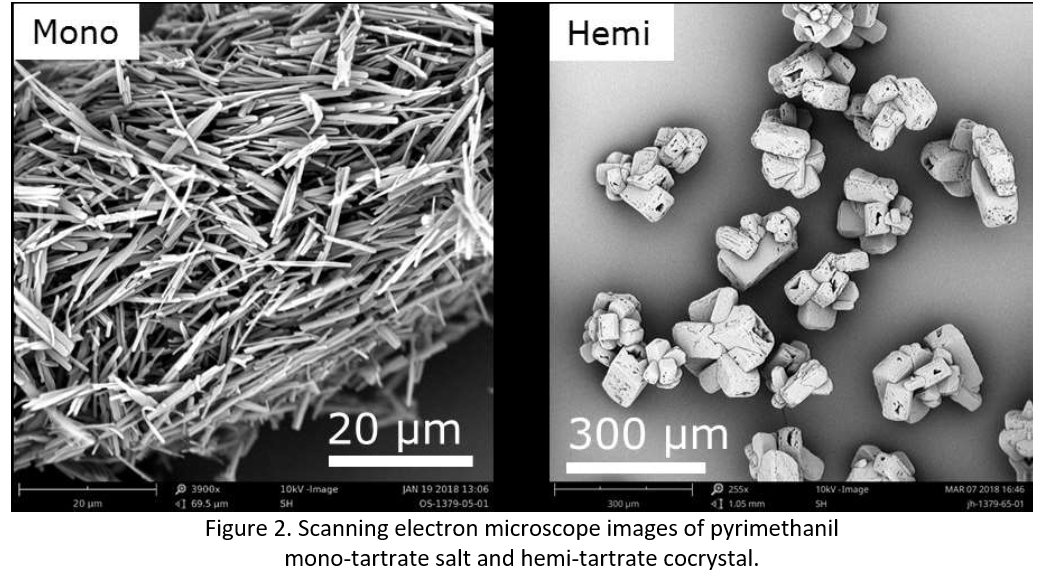
Optimizing a crystallization process typically involves a twofold approach. First, the parameters for preferentially crystallizing the desired form are identified. In this case the mono form was targeted as it was considered the most challenging based on the particle morphology (Figure 2). Second, the desired outcomes are targeted (e.g. yield, purity, morphology). In this specific example, an unoptimized crystallization yielded material of fibrous morphology with poor filtration properties. To improve the morphology, modelling was performed, followed by a design of experiments study. This involved optimizing parameters such as: solvent selection, residence time, seeding, anti-solvent addition protocol, cooling and stirring rates and establishing their impact on morphology. Considering these alterations, it was possible to improve the crystal morphology of the pyrimethanil mono-tartrate salt from a fibrous material to one with more desirable morphological properties (see Figure 3).
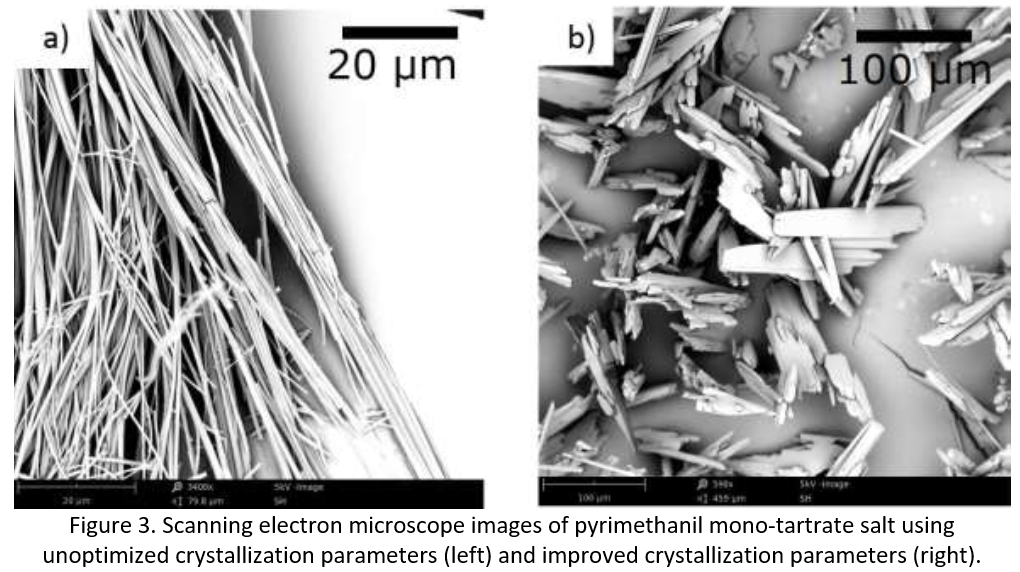
The multi-component crystallization journey
Finding the appropriate multi-component solid form of a pharmaceutical or agrochemical is a journey of discovery, development and optimization. The path to commercialization involves working closely with a client to establish exactly what properties are essential and where potential compromises can be taken. This makes working with experts who understand and operate in a flexible, bespoke approach essential to ensure the delivery of a large scale successful synthesis for any project.
Authors:
Suzanne Buttar, Head of Solid State Services; Thierry Bonnaud; Team Leader; Osama Suleiman, Team Leader; Kate Wittering, Senior Scientist; Joe Harris, Crystallization Scientist; Chloe Bartlet, Analytical Scientist at Johnson Matthey